Published by Alberto BORETTI, Department of Mechanical Engineering, College of Engineering, Prince Mohammad Bin Fahd University, Al Khobar, Saudi Arabia
Abstract. Data of energy economy of battery electric vehicles without a range extender internal combustion engines (BEV) and with a range extender internal combustion engine (BEVx) are reviewed and integrated with simulations by models. A BEV with an on-board, high efficiency, electricity generator based on a positive ignition (PI) internal combustion engine (ICE) is then proposed as a way to improve the uptake of the BEV improving their range and performance as well as their economic and environmental impact. The small ICE, that is working continuously, stationary, fixed load and speed, and the generator similarly optimized for a single point operation, permit an efficiency fuel chemical-to-electric approaching 50%. This is much better than producing electricity centralized from combustion fuels (average efficiency with included distribution and recharging losses at about 30%), and it does not require any electric recharging infrastructure. Simple but reliable extrapolations from the production BEV and BEVx of different battery capacity on the same vehicle platform, plus the simulations, demonstrate that this BEVy may deliver miles-per-gallon (MPG) working gasoline 13% better than any present plug-in-hybrid-electric-vehicle (PHEV) currently available, and MPGe (MPG-equivalent) working electric 12% better than the existing BEV on the same platform with a larger battery pack and no range extender, or 27% better than the BEVx on the same platform with a larger battery pack and range extender. Finally, this BEVy may permit a range over 600 miles with 10 gallons of gasoline onboard, in line with the best PHEV currently available.
Streszczenie. W artykule analizowano możliwości wykorzystania wewnętrznego silnika spalinowego o dużej efektywności do ładowania akumulatora. Możliwości te analizowano dla różnych modeli samochodu. Porówanno też tego typu rozwiązanie z samochodami hybrydowymi. (Możliwości ładowania alkumulatora samochodu elektrycznego przy wykorzystaniui wewnętrznego silnika spalinowego)
Keywords: battery electric vehicle; range extender; internal combustion engine; energy efficiency; on-board electricity production
Słowa kluczowe: akumul;ator samochodowy, silnik spalinowy, ładowanie akumulatora.
Introduction
A Battery Electric Vehicle (BEV) is a vehicle powered by an electric motor/generator that is connected to a rechargeable battery pack. The latest trends in the development of novel BEVs is towards the use of much larger battery packs. This increases the economic and environmental costs of producing, using, and disposing of the batteries [1]. Additionally, larger battery packs increase the weight of the vehicle, reducing performance, and increasing energy consumption [1]. Finally, as the materials needed for the battery components are in short supply [1] and in some cases their mining is unethical, this creates sustainability and ethical issues.
The share of renewable energy is increasing, but still relatively modest [2]. The latest statistic of the world Total Primary Energy Supply (TPES) assigns wind and solar, plus other minor renewables, a total of 1.7%. Wind and solar energy, this latter almost exclusively photovoltaic, are only available when the resource is available. When there is no wind or sun energy resource, then there is no electricity production. This problem can only be solved with huge energy storage, that in addition to pumped hydro where possible, it is again a problem of producing other batteries [3], [4].
Solar photovoltaic is presently the cheapest renewable energy. It is only available during daylight time. The capacity factor of solar photovoltaic plants is about 0.3. Every day, they are zero during nighttime, then something up to maximum unity during the day, depending on the day, following seasonal variability, and weather conditions such as clouds and rain.
For a solar photovoltaic plant of power P MW, what is needed is a battery of actual power roughly 0.3·P MW, and actual energy minimum 12·0.3·P MWh, as there are on average 12 hours of the day and 12 hours of the night in a day. This is a huge demand for present technology batteries, as the world’s largest battery, the Hornsdale power reserve battery has nominal storage capacity (energy) of only 185 MWh and nominal capacity (power) of 100 MW. It is never charged or discharged at more than one half of the nominal power, and it is never charged or discharged of more than one half of the nominal energy.
The electricity produced centralized by burning fossil fuels is done with fuel conversion efficiencies fuel-chemical-to- electric below 33% [5]. Then, there are the distribution losses, as well as the losses charging the batteries and within the batteries of the electric vehicles when charged. Additionally, there is a need to build a recharging infrastructure. As internal combustion engines have surpassed the 50% fuel conversion efficiency [6], it makes sense to produce electricity onboard on-demand. This also brings the advantage of no distribution and charging and discharging losses, plus no need for a recharging infrastructure.
Thus, it makes sense to design BEV with smaller rather than larger battery packs, and adopt on-board electricity production by high-efficiency internal combustion engines (BEVy hereafter).
The high-efficiency internal combustion engine (ICE), drives an electric generator at a constant speed and load continuously recharging the battery. The battery is discharged or recharged by the motor/generator following the road load.
This concept is different from BEV with a range extender (BEVx hereafter). In BEVx such as the BMW i3 Rex with a range extender engine, electricity is only produced in specific circumstances by a low-efficiency engine to permit reaching the nearest recharging station for the battery.
The BMW i3 Rex is a B-class hatchback, with an electric motor-generator on the rear wheels. The transmission is single-speed. The battery pack is underfloor Li-ion. The range-extender gasoline engine is optional to the baseline BEV.
The engine is in the specific case a low-cost, low-tech motorcycle engine converted for the purpose that is switched on and off continuously during a cycle covered with the battery almost empty. Electricity may only be produced when the battery state-of-charge falls below a very low charge threshold, with the ICE then switched off immediately after a second close threshold is reached. This is a very inefficient way to use fuel energy.
The design of BEVx has been focused so far on low weight and cost, compact design, good noise, and vibration harshness (NVH) behavior. However, being fuel energy inefficient, such design does not provide any advantage versus the use of BEV with large batteries. The opposite is the situation of the proposed BEVy.
Both the proposed BEVy and a BEVx are series-hybrid vehicles, where the ICE may only drive a generator charging the battery or passing through the motor/generator fitted to the wheels.
ICEs for F1 racing applications have surpassed fuel conversion efficiencies of 50% in transient operation, [1], [6], [7], [8], [9]. About the same fuel conversion efficiencies above 50% were also previously obtained in compression ignition (CI) diesel ICEs for FIA WEC LMP 1 application [8]. Large low-speed CI ICEs, for power generation and marine applications, have achieved fuel conversion efficiencies even larger, also approaching 55%, since the end of the last century [9], [10], [11]. Thus, it is within easy reach to design ICEs that are working stationary driving a generator similarly optimized for constant speed and load, of efficiency fuel-chemical to electric approaching 50%.
Worth to mention, the average power of driving cycles is usually quite small, thus also this ICE can be designed for relatively small power, and be very compact and of extremely reduced weight.
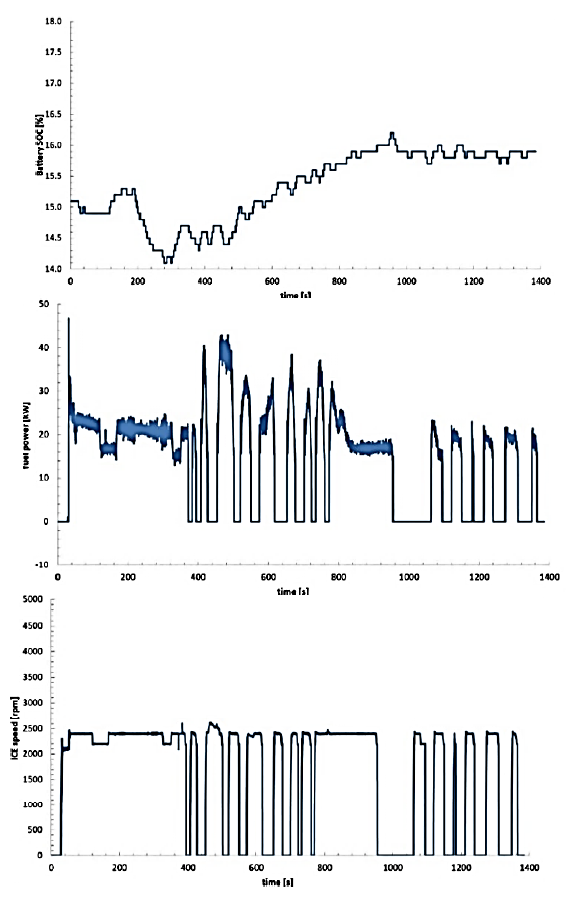
Test by Argonne National Laboratory (ANL) [12] of a BMW i3 REX with range extender shows the limit of the BEVx design. The low tech engine derived from a motorcycle engine is able at the most of fuel conversion efficiencies fuel-chemical-to mechanical in the mid 30%. The engine gets switched ‘on’ and ‘off’ many times in a cycle. It is ‘on’ when the state-of-charge of the battery (SOC) falls below a very low threshold, and it is switched ‘off’ immediately after when a marginally higher SOC is reached [13]. This way, the efficiency fuel-chemical to electric is reduced to 30% and even below, depending on the specific driving cycle. One sample operation of the engine over a cycle covered wth battery almost empty is provided in Fig. 1 (images reproduced modified after [13]).
Both the ICE and the generator that is driven by this engine may work much better if they are operated at a single speed and load continuously, and if a high tech design of the ICE is adopted, the conversion efficiency fuel-chemical-to-electric can approach 50%. Operating at a constant speed and load over a much wider range of battery SOC, for example from 30% charge to 90% charge, this high-efficiency engine and generator optimized to work at a constant speed and load may beat the energy efficiency of the distributed centralized power generation by combustion fuels [14].
A comparison of the BMW i3 Rex to the best plug-in hybrid electric vehicles (PHEV) for range and energy economy is shown in Fig.2. The figure presents the MPG (miles-per-gallon) working gasoline, MPGe (miles-per-gallon equivalent) working electric depleting the battery, and the range, of commercial plug-in hybrid electric vehicles (PHEV) featuring an ICE, a traction battery, and an electric motor-generator. These PHEVs include series hybrids, parallel hybrids, and more complex series/parallel hybrids.
Additionally to Fig.2, also Table 1 compares the MPG and MPGe of the BMW i3 with and without range extender (i.e. a BEV and a BEVx) and the Toyota Prius Prime (a PHEV) for the MY2020. Working gasoline, a 2020 Toyota Prius Prime has an MPG of 54 miles. The 2020 BMW i3 with Range extender has only 31 miles. This is a huge difference. Worth to note is also the much better energy efficiency working electric depleting the battery, which is 133 MPGe for the 2020 Toyota Prius Prime, and it is 113 MPGe for the 2020 BMW i3 without Range extender, or even 100 MPGe for the heavier 2020 BMW i3 with Range Extender.
Focusing on the previous MY, the 2019 BMW i3 Rex 120 Ah battery has an MPG/MPGe of only 0.31. The 2019 Toyota Prius has 0.42. The range of gasoline over electric of a 2019 Toyota Prius is 24, but it is only 0.57 for the 2019 BMW i3 Rex. While the 2017/2018 Toyota Prius has an MPG of 54 miles, and an MPGe of 133 miles, the 2019 BMW i3 Rex 120 Ah battery has an MPG of only 31 miles and an MPGe of only 100 miles. By drastically reducing the weight, as shown by the BMW i3 Rex 94 Ah, the MPG increases to 35 miles, and the MPGe increases to 109-111 miles. The Toyota Prius has only 25 Ah (MY2017/2018) batteries, a much more sophisticated engine, plus a much more complex hybrid driveline.
From Fig. 2 and Table 1, it is clear as the energy density of batteries is a major issue, the same as the low tech engine employed inefficiently in the optional range extender. A BMW i3 Rex (with range extender) has an MPGe of 117 with the 60 Ah battery (MY2014/2016), 111 with the 94 Ah battery (MY2017/2018), and only 100 with the 120 Ah battery (MY2019/2020). A BMW i3 without range extender has an MPGe of 124 with the 60 Ah battery (MY2014/2017), 118 with the 94 Ah battery (MY2018), and only 113 with the 120 Ah battery (MY2019/2020).
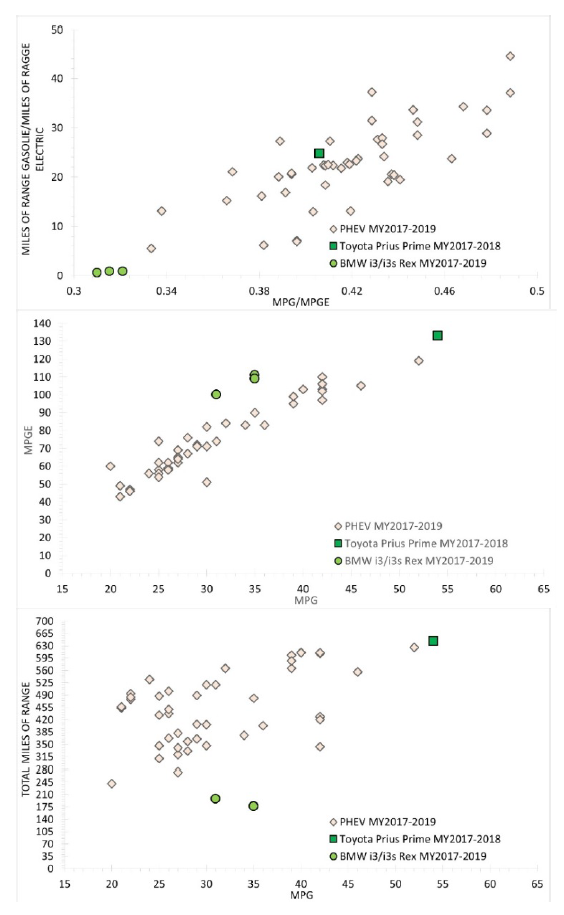
Table 1 – Latest fuel economy data of a 2020 BMW i3, without and with range extender, and of a Toyota Prus Prime. Image reproduced modified from http://www.fueleconomy.gov. The MSRP of the BMW i3 with range extender is $48,300. The MSRP of the BMW i3 without range extender is $44,450. The MSRP of the Toyota Prius Prima is $27,600 to $33,500.
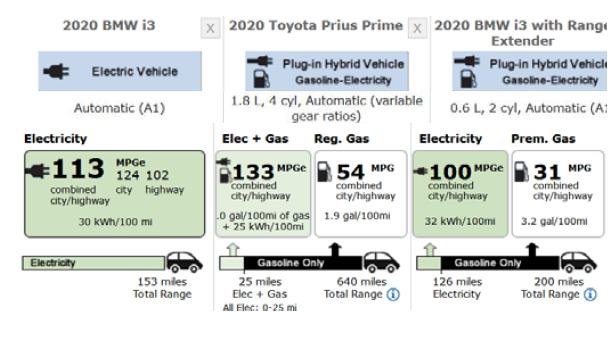
These differences of MPG/MPGe are a clear indication of the energy efficiency penalty because of the weight penalty adopting larger batteries with everything else unchanged.
The range using the chemical energy of the gasoline fuel is much larger than the range using the battery. This is because the storage of energy on-board is much easier by a combustion fuel such as gasoline or diesel.
A Toyota Prius, that is a complex hybrid PHEV with only a small traction battery, and opportunity to propel the vehicle on battery, on the engine and both, and also recharge the battery by using the engine, in addition to regenerative braking, has both MPG and MPGe much larger than a series hybrid BMW i3 Rex with a large battery pack and the constraint to use the small engine only to recharge the battery when it is almost depleted to reach the nearest recharging station.
The energy density of present technology batteries (Li-Ion) compared to the energy density of transportation fuels is still small. The energy density of batteries has been dramatically improved, but it is still more than one order of magnitude less of what it should be. Present technology batteries are also not environmentally friendly and their production and disposal create many issues. Moreover, the materials needed for the batteries of the cars and the energy storage for a grid that is feed by wind and solar only, are in shorter supply than the combustion fuels. Even if we only focus on the CO2 emission, there is no advantage.
Gasoline has 43.5 MJ/kg and 32 MJ/liter. Diesel has 42.6 MJ/kg and 36 MJ/liter. Li-ion batteries still have 0.63 MJ/kg and 1.08 MJ/liter. Thus, to have onboard a significant amount of energy by batteries translates into an unaffordable volume and an even less affordable weight, compared to gasoline and diesel. With the proposed high-efficiency electricity production on-board, almost 50% of this energy is converted to electricity when needed. This translates into a huge advantage.
Fig.2 and Table 1 indicate the need to revise the idea behind BEV with range extender (BEVx) that must become BEV with high-efficiency on-board electricity production (BEVy) to be competitive with the BEV of large batteries.
The energy economy data here shown of BEV and PHEV (also including BEVx) is proposed in [15] and [16]. The energy economy is given for the US certification cycles.
The proposed BEVy design, where electricity is generated on-board of the vehicle by using a high-efficiency ICE working continuously in the best point of operation and recharging the battery continuously, permits to store energy on-board as gasoline fuel in the tank much more efficiently than with additional battery packs fully charged.
There is an order of magnitude difference between the energy density of combustion fuels such as gasoline or diesel and the energy density of Li-ion batteries. This makes a string case for novel BEVy.
Method
The fuel economy data of BMW i3/i3s BEV and BEVx of different battery size and weight have been analyzed in [14] and [17] to derive a relationship between the energy efficiency over driving cycles and the weight of the vehicle. Details of the extrapolation procedure are proposed in [14] and [17].
Simulations were also performed with a computer-aided engineering tool, as well as with a simple Newton’s equation model to show as the energy consumption of an electric car of a given aerodynamic and rolling resistance over a given cycle is a linear function of the weight.
As shown in [18], if the efficiency of the energy conversion and transfer battery-to-wheels is ηbw (propulsion) and the efficiency of the energy conversion wheels-to-the battery is ηwb (regenerative braking), then the electric energy out of the battery can be approximated as:
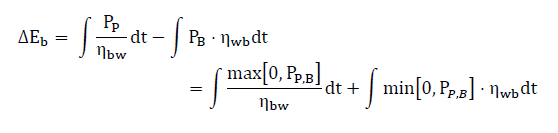
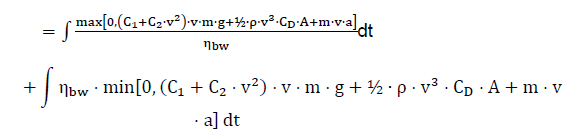
In this equation PP is the propulsive power, PB is the braking power, t is the time, v the velocity, given as a function of t, C1, and C2 are two coefficients expressing the rolling resistance, m is the mass of the vehicle, g the gravity acceleration, ρ is the air density, CD is the drag coefficient for the reference area A, and a is the acceleration, i.e. the known derivative of the velocity in time.
If we disregard the efficiency variations, the electric energy out of the battery for a given vehicle is linearly proportional to the mass of the vehicle.
Results
The benefits of the proposed BEVy design are discussed in [14] and [17] for passenger car applications.
A racing car application, a Le Mans hypercar, is also covered in [17]. It is shown in [17] as the use of the most of the hardware of an Audi R18, only adopting a motor-generator of the total permitted power 550 kW on the rear wheels, rather than the smaller motor-generator of reduced power on the front wheels, and replacing the mechanical transmission with a generator, this series hybrid car can be 10 seconds per lap faster, or permitting more laps before refueling with same onboard fuel if operated at about same lap time of the original Audi R18.
In the below results, the platform considered is always the BMW i3. The extrapolated performance values for the proposed BEVy are obtained from the data shown of production BMW i3/i3s BEV and BEVx with different battery capacity, power, and fuel tank.
The proposed BEVy has given power and a given fuel tank. It is also assumed an efficiency chemical-to-electric of about 49%. The extrapolation formulae are discussed in [14] and [17].
As shown in [17], the MPGe vs. weight of existing BEV and BEVx and the proposed BEVy of different fuel tank capacity may be approximated by the linear relation (y=MPGe, x=weight in lbs) y = -2.61E-02·x + 1.95E+02. The linear fitting has R² = 8.86E-01. This means a BEVy of weight 2567 lbs with on-board 0.96 liters of gasoline, has an MPGe of 128 miles when working on battery. By increasing the amount of fuel on-board to 10 gallons, the weight only increases to 2621 lbs (6 lbs per extra gallon of gasoline). The MPGe is almost unaffected at 127 miles.
This is the operation working on battery. Working gasoline, as shown in [17], while the MPG of existing BEVx varies between 35 and 43 miles, depending on the heavier or lighter arrangement (i.e. larger or smaller battery), the MPG of the proposed BEVy of different fuel tank capacity may be approximated by the linear relation (y=MPG, x=weight in lbs): y = -0.0126·x + 95.06. This is the MPG working electric with a fuel-chemical to the electric conversion efficiency of 0.49.
Thus, the BEVy with onboard 0.96 gallons of gasoline has an MPG of 63 miles, and the BEVy with onboard 10 gallons of gasoline has about the same MPG of 62 miles.
Worth to mention, the weight of a vehicle with a fuel tank reduces when the fuel is consumed, opposite to the weight of a vehicle with a battery that does not reduce its weight when the battery runs empty.
Fig. 3 presents the range vs. weight of existing BEV and BEVx and the proposed BEVy of different fuel tank capacity on the BMW i3 platform. Fig. 4 summarizes the results for the proposed BEVy with a 10 gallons fuel tank compared to the PHEV of Fig.2. All the data of existing BEV and BEVx produced on the BMW i3 platform are finally proposed in Table 2, together with modelled values of variants also including the proposed BEVy.
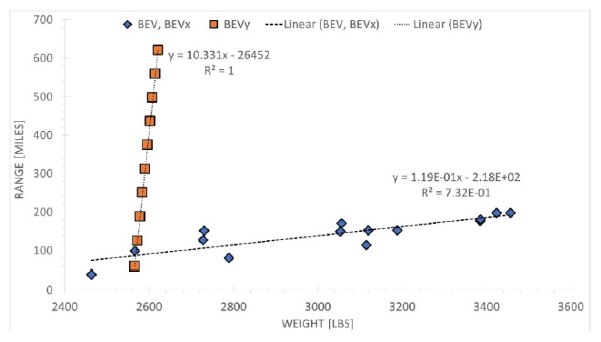
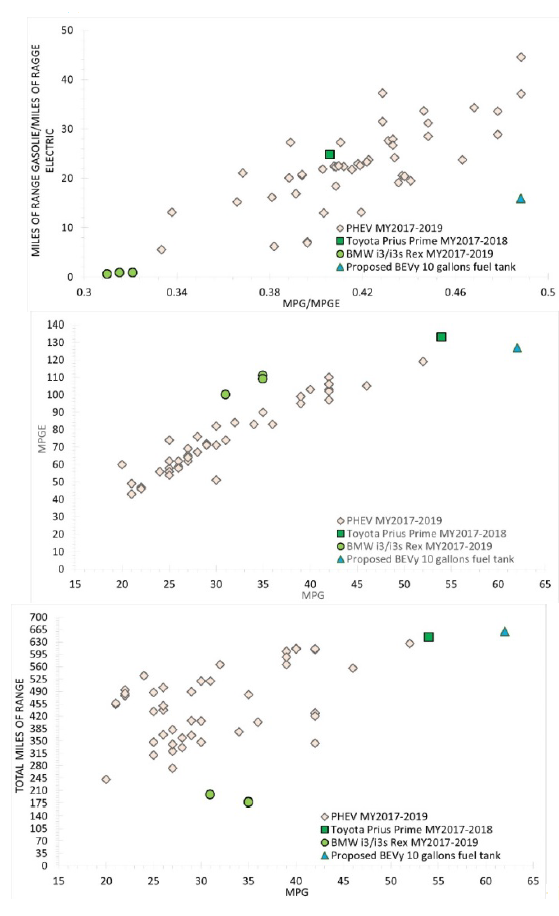
Table 2 – Summary table of energy economy, battery size and weight of existing BMW i3 BEV and BEVx and proposed BEVy on same platform. Energy economy values are EPA values except those with (*) that are modelled values. Table reproduced modified from [17].
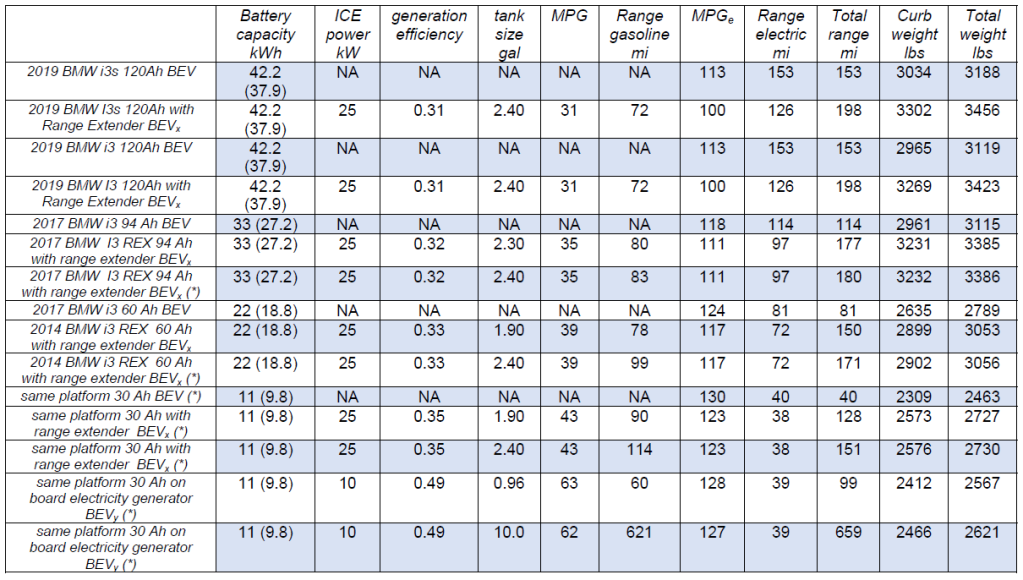
The range of a BEV or BEVx increases minimally with the weight. It is about (y=range, x=weight in lbs) y = 1.19E- 01·x – 2.18E+02. The linear fitting has R² = 7.32E-01.
A BEVy of 30 Ah battery may permit, on the same platform of the BMW i3, a range of 40 miles without the onboard electricity generator, and a range of 39 miles with the onboard electricity generator. The MPGe combined city/hwy is 130 and 127 miles respectively.
The BEVy MPGe exceeds by 12% the MPGe of the 120 Ah 42.2 kWh battery capacity BEV without range extender and of 27% the MPGe of the 120 Ah 42.2 kWh battery capacity BEVx with range extender. The MPG is 60 miles. The range is 59 miles with onboard gasoline storage of 0.96 gallons.
This MPG working gasoline is 13% better than any present plug-in-hybrid-electric-vehicle (PHEV) currently available, reaching the most 54 MPG.
The MPG/MPGe of the BEVy is the 0.49 efficiency of electricity production on board. The range of gasoline vs. electric can be easily increased by increasing the fuel onboard.
With an MPG well above 60 miles, the proposed BEVx has a better fuel energy efficiency than every other PHEV. The MPGe is still less than the 133 miles claimed for a Toyota Prius MY2017 and MY2018. The proposed BEVy with a 10 gallons fuel tank full has also a total range above a Toyota Prius MY2017 and MY2018.The proposed BEVx has advantages in terms of MPG and range vs. a Toyota Prius despite the much simpler series hybrid design.
The battery Ah to kWh conversion formula used in Table 2 is kWh = (Ah·V) /1,000 where V is the voltage in volts. The 120 Ah 42.2 kWh battery has actual energy stored 37.9 kWh, the 94 Ah 33 kWh battery has actual energy stored 27.2 kWh, the 60 Ah 22 kWh battery has actual energy stored 18.8 kWh, and the 30 Ah 11 kWh battery has actual energy stored 9.8 kWh. Additionally, for longer life, the battery is never discharged of the full actual energy.
Summary/Conclusions
The proposed series-hybrid with a small, high-efficiency internal combustion engine working constant speed and load to drive a high-efficiency generator recharging a small battery, referred to as BEVy in the paper, has significant advantages. The MPG, MPGe, and especially range are dramatically improved vs. existing BEV and range-extender BEV, referred to as BEVx in the paper.
Considering the batteries are presently dragging down the progress of the electric vehicles, being an economic and environmental burden, also in consideration of the lack of infrastructure, the still minimal contribution by renewables to the total primary energy supply, and the less efficient production by fossil fuels of the electricity to be distributed, the use of a small battery pack and high-efficiency electricity production on board appears to be the way to progress towards widespread electric mobility.
The proposed vehicle adopting the platform of a BMW i3, with a small 30 Ah 11 kWh battery pack, but the high-efficiency on-board 10 kW ICE electricity generator, and onboard gasoline storage of 0.96 gallons, may allow a range of 39 miles electric only, with MPGe combined city/hwy of 127 miles. The MPG is 62 miles, while the range is 59.5 miles. This MPG is 13% better than any present PHEV currently available in the market.
Small increments of the fuel tank capacity translate in large improvements of the range with minimal reduction of the energy economy. A 4 gallons’ fuel tank would allow more than 220 miles of range. A 10 gallons’ fuel tank would permit the same range of best PHEVs, at 660 miles total, 39 miles electric, and 621 gasoline.
While the end of the internal combustion engine may have been already decided [19], as already decided is the end of the production of the BMW i3 rex with range extender [20], it makes a lot of sense for the economy and the environment, as well as the sustainability of transport and power generation, to support solutions such as the proposed BEVy.
This is in between the best options available for mass mobility within a time frame of one to two decades.
REFERENCES
[1] Boretti, A., “Life cycle analysis comparison of electric and internal combustion engine-based mobility,” SAE Technical Paper 2018-28-0037, 2018.
[2] International energy agency (IEA), “Key World Energy Statistics (2018),” webstore.iea.org/key-world-energy-statistics-2018, accessed January 1, 2020.
[3] Boretti, A. and Castelletto, S., Cost of wind energy generation should include energy storage allowance, Scientific Reports, 10:2978, 2020. doi.org/10.1038/s41598-020-59936-x
[4] Boretti, A., Energy storage needs for an Australian National Electricity Market grid without combustion fuels, Energy Storage. First Published: 6 October 2019. doi.org/10.1002/est2.92
[5] Energy Information Administration (EIA), “Average Tested Heat Rates by Prime Mover and Energy Source, 2007 – 2017,” http://www.eia.gov/electricity/annual/html/epa_08_02.html, accessed January 1, 2020.
[6] Boretti, A., “Transient positive ignition internal combustion engines have now surpassed the 50% fuel conversion efficiency barrier,” International Journal of Hydrogen Energy, 44(14):7051-7052, 2019.
[7] Boretti, A., “Progress of Direct Injection and Jet Ignition in Throttle-Controlled Engines,” SAE Technical Paper 2019-26-0045, 2019.
[8] Boretti, A., “Advances in Turbocharged Racing Engines,” (Warrendale, SAE International, 2019). ISBN 978-0-7680-0014-6, 236 pages.
[9] Noble, J., “Mercedes F1 engine hits ‘remarkable’ efficiency target on dyno,” http://www.autosport.com/f1/news/131772/mercedes-engine-hitsremarkable-dyno-target, accessed January 1, 2020.
[10] MAN Diesel & Turbo, “LNG Carrier Propulsion by ME-GI Engines and/or Reliquefication,” http://www.idmeb.org/contents/Resource/LNG_Propulsion_02_29_38.pdf, accessed January 1, 2020.
[11] MAN Diesel & Turbo, “LNG Carriers with ME-GI Engine and High-Pressure Gas Supply System,” marine.mandieselturbo.com/docs/librariesprovider6/technicalpapers/lng-carriers-with-high-pressure-gas-supplysystem.pdf?sfvrsn=16, accessed January 1, 2020.
[12] Argonne National Laboratory (ANL), “2014 BMW i3 EV,” http://www.anl.gov/es/energy-systems-d3-2014-bmw-i3rex, accessed January 1, 2020.
[13] Boretti, A., “Analysis of the energy storage battery and fuel tank of a Commercial Electric Vehicle with Range Extender during Charge Sustaining Operation,” Energy Storage, e129, 2020. doi.org/10.1002/est2.129
[14] Boretti, A., “Electric vehicles with small batteries and high-efficiency on-board electricity production,” Energy Storage 1(4), e75, 2019.
[15] FuelEconomy.gov, “Compare Electric Cars Side-by-Side,” http://www.fueleconomy.gov/feg/evsbs.shtml, accessed January 1, 2020.
[16] FuelEconomy.gov, “Compare Plug-in Hybrids Side-by-Side,” http://www.fueleconomy.gov/feg/phevsbs.shtml, accessed January 1, 2020.
[17] Boretti, A., “Series BEV with a small battery pack and high-efficiency ICE onboard electricity production: B-class, high-roof hatchback and Le Mans Hypercar applications”, SAE P. 2020-01-2250, 2020.
[18] Boretti, A., “Battery electric vehicles with small battery pack and high efficiency onboard electricity production by a combustion engine”, FISITA2020 submitted paper under review.
[19] Ernst, J., “Volkswagen says last generation of combustion engines to be launched in 2026,” http://www.reuters.com/article/us-volkswagen-emissionscombustion/volkswagen-says-last-generation-of-combustionengines-to-be-launched-in-2026-idUSKBN1O32O6, accessed January 1, 2020.
[20] Autocar, “BMW to cease production of the i3 Range Extender,” http://www.autocar.co.uk/car-news/new-cars/bmw-ceaseproduction-i3-range-extender, accessed January 1, 2020.
[19] Boretti, A. and Castelletto, S., Cost of wind energy generation should include energy storage allowance, Scientific Reports. Scientific Reports, 10:2978, 2020. doi.org/10.1038/s41598-020-59936-x
[20] Boretti, A., Energy storage needs for an Australian National Electricity Market grid without combustion fuels, Energy Storage. First Published: 6 October 2019. doi.org/10.1002/est2.92
Authors: Prof. Dr. Alberto Boretti, Office No.: S-022, Prince Mohammad Bin Fahd University, P.O. Box 1664, Al Khobar 31952, Kingdom of Saudi Arabia. Phone: +966-13-849-4367 email: a.a.boretti@gmail.com
Source & Publisher Item Identifier: PRZEGLĄD ELEKTROTECHNICZNY, ISSN 0033-2097, R. 96 NR 12/2020. doi:10.15199/48.2020.12.16